Surface-enhanced Raman Spectroscopy (SERS) Sensing of Bioanalytes
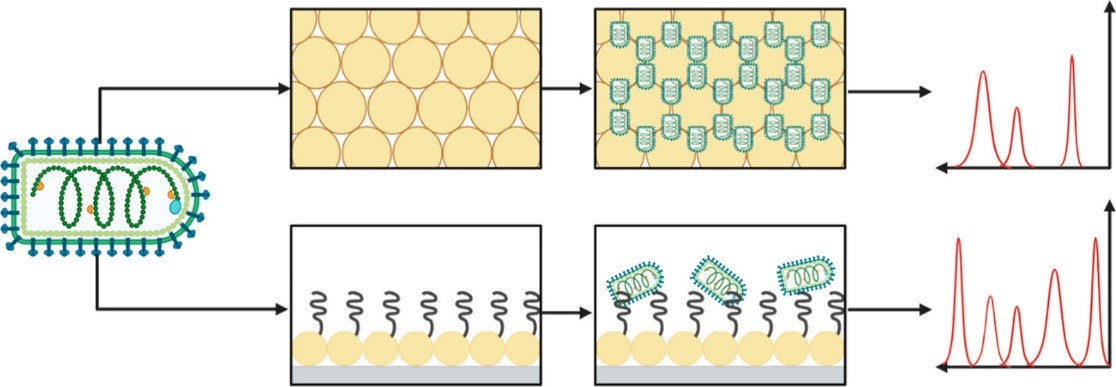
The Haynes group has a long history of research in SERS, especially in the application of SERS for biosensing, and focuses on two areas: substrate design and signal transduction mechanisms. Our group works on multiple types of substrates, such as metal film-over-nanospheres (MFONs) and nanoparticle colloids, to develop inexpensive and simple substrates with high SERS enhancement factors without requiring advanced lithographic techniques. Current work in the group focuses on synthesizing chiral gold nanoparticles as well as silica-coated gold nanorods to detect specific analytes with high sensitivity. We also investigate different methods to facilitate signal transduction, such as using affinity agents with physical or chemical affinity for the analyte to bring analytes closer to the high enhancement region of SERS substrates or using the structure of a substrate to physically trap analytes within the enhancement region. Current work in this area includes the use of linear polymers as affinity agents for a wide range of analytes such as different strains of bacteria and using nanogaps in MFON substrates to trap and detect virus-like particles. Additionally, our group employs machine learning techniques such as principal component analysis (PCA) and support vector machines (SVM) to aid in data analysis and classification of samples from their Raman spectra.
Design of Silica Nanoparticles for Sustainable Agriculture
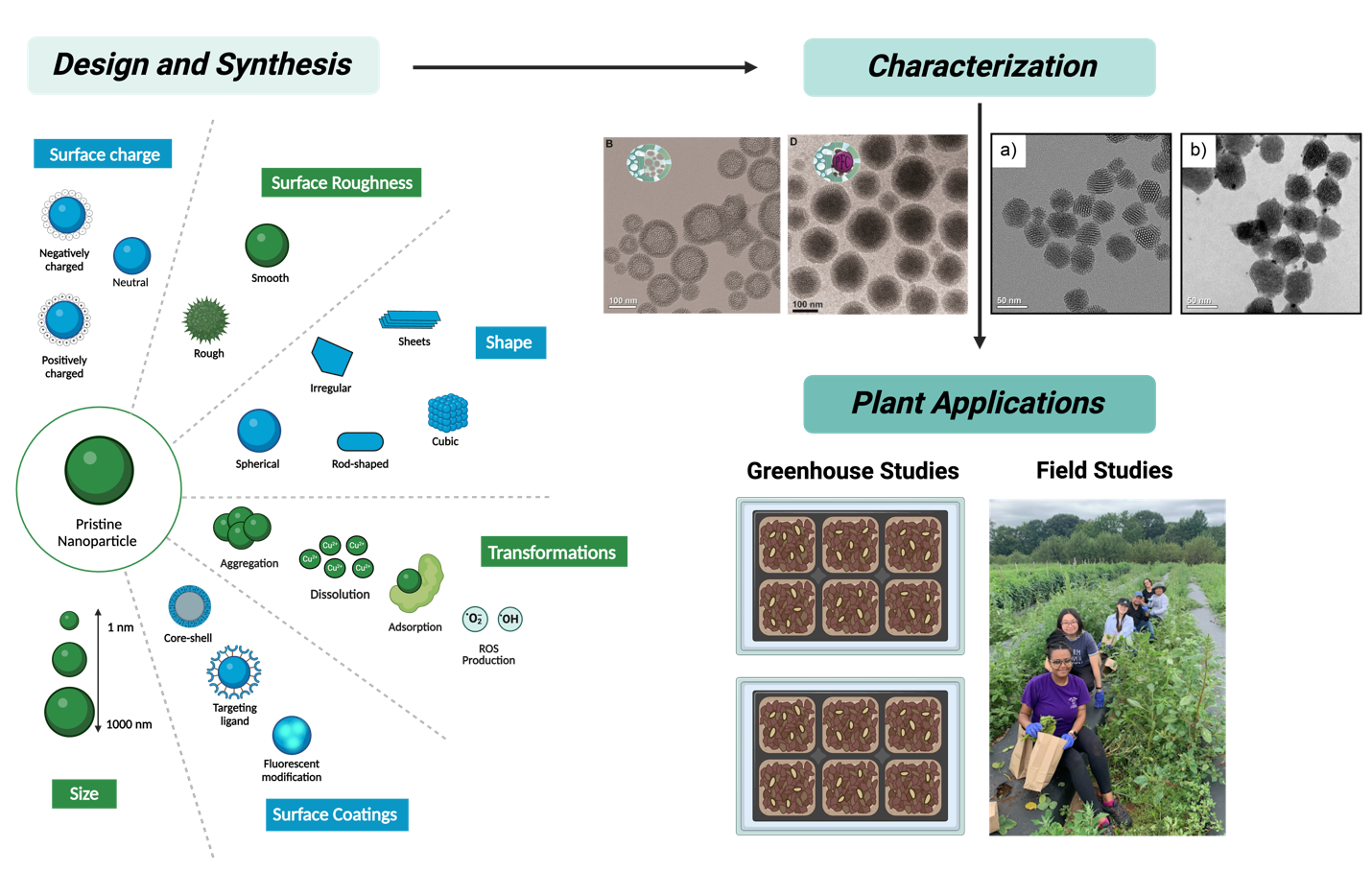
Silica nanoparticles have been previously used in the Haynes group for a range of biomedical applications. In recent years, our work has shifted towards leveraging our knowledge from biomedicine towards designing, synthesizing, and characterizing silica-based nanoparticles for agriculture and other applications. We use our chemical expertise to tune various nanoparticle characteristics such as size, porosity, dissolution rate, surface charge, and functionalization with the overall goal of measuring their impact on different plant models. Our projects have focused on tuning the dissolution of silica nanoparticles for applications in watermelon as well as coating porous silica nanoparticles with chitosan for applications in watermelon and soybeans. We are also interested in using responsive nanoparticles as delivery agents for various cargo. The Haynes group employs various nanoparticle synthesis methods as well as numerous characterization techniques such as dynamic light scattering, nitrogen physisorption, transmission electron microscopy, energy dispersive spectroscopy, confocal microscopy, and X-ray photoelectron spectroscopy, among others. Many of our projects in this area are collaborations with scientists at a range of academic, government, and commercial institutions. Overall, our work is contributing towards nano-enabled agricultural applications that can increase crop production and reduce plant disease progression to strive towards meeting UN Sustainable Development Goals.
Microelectrochemistry Sensing
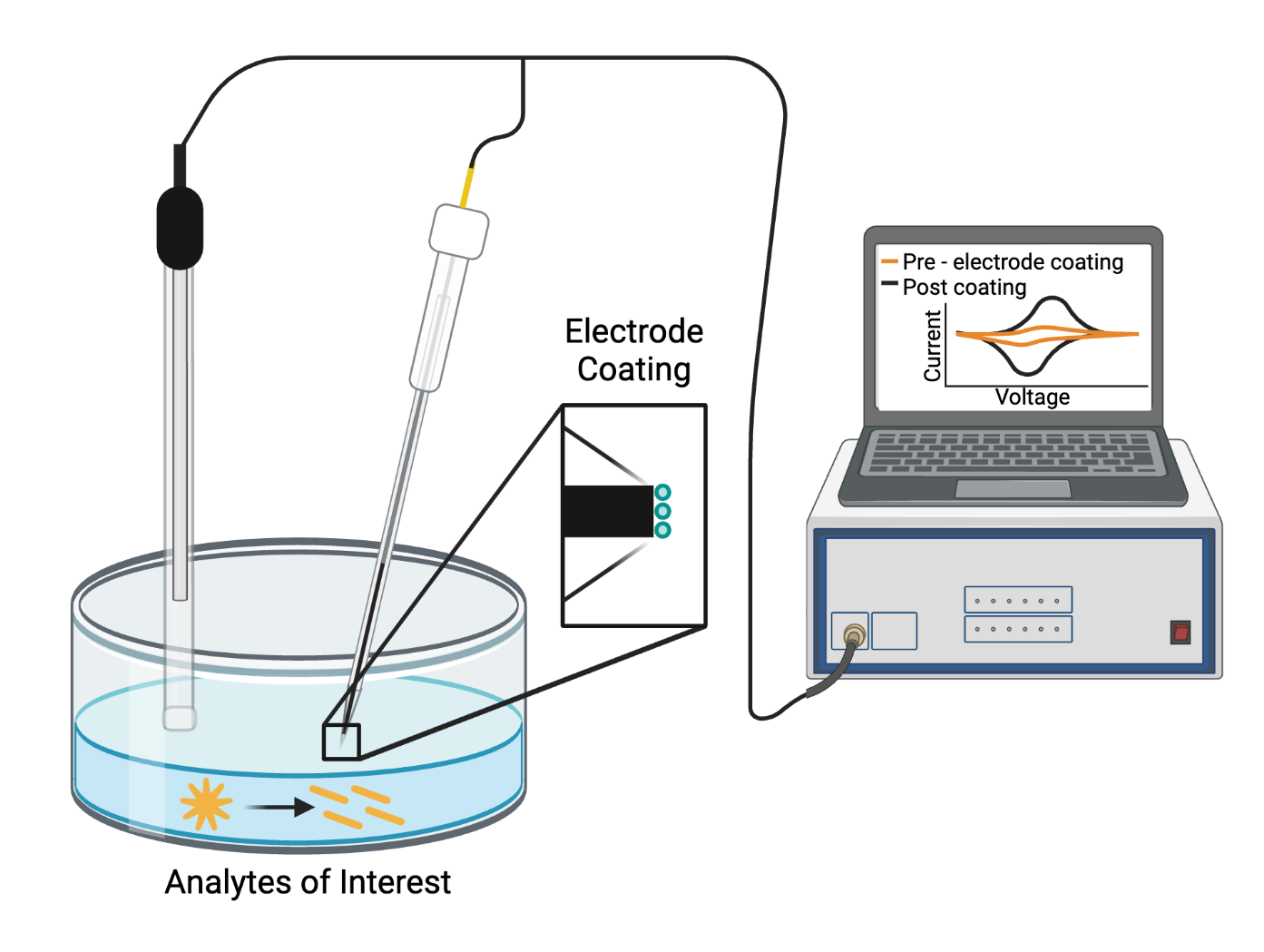
The Haynes Lab has been active in analytical electrochemistry since its very start, specifically micro-electrochemistry. Rather than large electrodes, we use in carbon-fiber microelectrodes. Made using glass capillary tubes and carbon-fiber, these microelectrodes allow electrochemical detection at the single-cell level. This means that they provide higher spatial and temporal resolution compared to typical electrode systems, with the ability to measure extracellular and intercellular analytes of interest. Past studies have focused on understanding cell platelet opioid receptors, tracking serotonin levels in sickle cell disease, and understanding changes in mast cell function in mice with and without malaria. Current studies focus on adapting carbon-fiber microelectrodes with different nanomaterial surface coatings to enhance their sensitivity for analytes of interest, including reactive oxygen species and neurotransmitters. We combine carbon-fiber microelectrodes and carbon dots to produce fluorescent, electrochemical sensors and exploit carbon-fiber microelectrodes to understand nanoparticle transformations. We can perform a wide range of electrochemical techniques from traditional cyclic voltammetry and chronoamperometry to fast scan cyclic voltammetry and single cell amperometry.
Carbon Dots: Fluorescent Nanoparticles for Analyte Detection
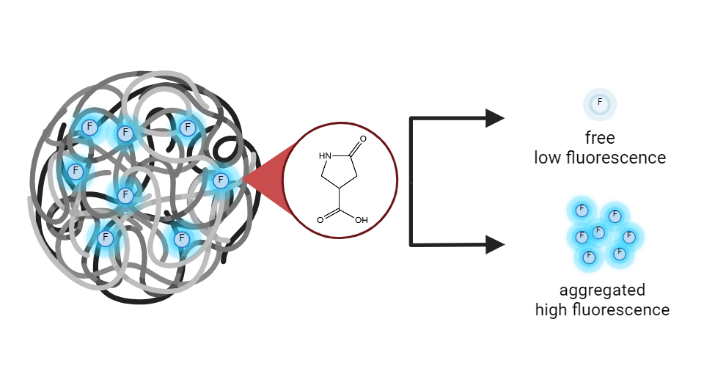
In recent years, carbon dots (CDs) have emerged as a new class of fluorescent nanoparticles that have piqued interest due to their facile synthesis, nontoxicity, tunable surface chemistry, and bright fluorescence. In the Haynes group, research has focused on synthesizing carbon dots for various applications while working to understand fundamental details of carbon dot formation and fluorescent mechanisms. Some CD-based work in our group includes using carbon dots for super-resolution imaging, identifying small molecule fluorophores that contribute to CD fluorescence, and understanding carbon dot toxicity and transformations.
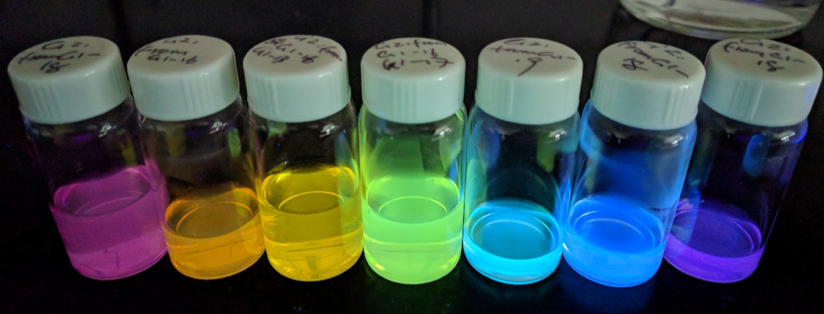
Current research with CDs in the Haynes group largely focuses on three real-world applications for carbon dots: using CDs to enhance phytoremediation of PFAS, developing CDs as a fluorescent sensor for foodborne pathogenic bacteria such as Salmonella, E. coli, and Listeria, and using CDs to coat electrode surfaces and enhance electrochemical signals for analyte detection. In all these projects, work is being done to further understand the structure and fluorescence of the CDs so that they can be efficiently used in a variety of applications.
Developing Nanoparticles for Per- and Poly-fluoroalkyl Substances (PFAS) Remediation
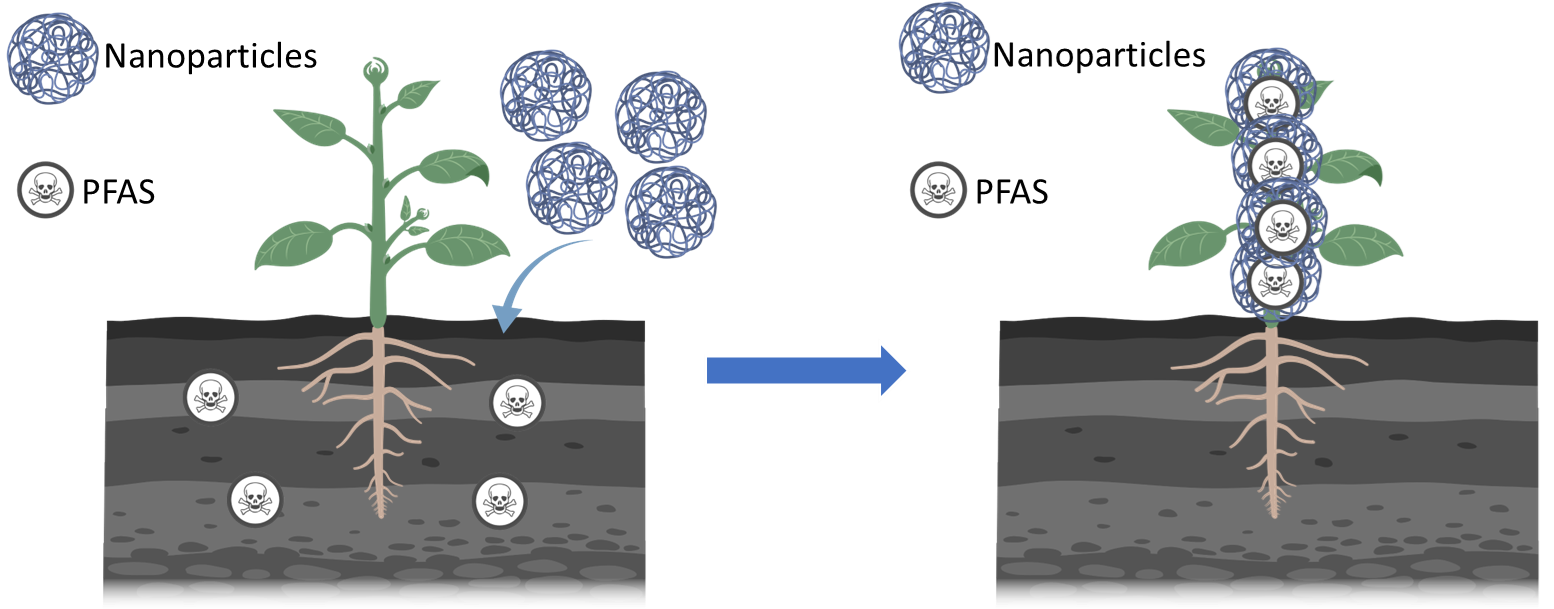
Concerns about per- and poly-fluoroalkyl substances (PFAS) have been raised globally as they are bioaccumulative, highly persistent, and present a range of health risks. Numerous studies have suggested phytoremediation as a sustainable PFAS remediation strategy; however, phytoremediation efficiency for PFAS is highly dependent on PFAS chain length, with limited uptake and removal of longer chain PFAS. The Haynes group has been working on developing carbon dots and porous silica nanoparticles as carriers to enhance the phytoremediation of PFAS from contaminated sites. The nanoparticles are designed and modified to have affinity for both short and long chain PFAS and have the potential to be taken up by plants. Different analytical methods such as 19F NMR and LC-MS/MS are used to monitor the interaction between the nanoparticles and PFAS. Plant experiments are also conducted in collaboration with other groups to assess and optimize the application of the nanoparticles in phytoremediation systems. With the successful completion of the studies, we will have an efficient and sustainable way to remove PFAS from environmental matrices, thus decreasing their bioaccumulation and exposure risk to humans. This project leverages our groups ability to synthesize nanoparticles for specific applications and develop analytical methods to probe and understand fundamental nanoparticle-small molecule interactions.